Subterranean detection using gravity
New capabilities enabled by quantum sensors
This post continues a series on quantum technologies.
The 2023 Defence Strategic Review has brought into sharp focus the Indo-Australian Archipelago. This chain of islands could be a difficult untrusted littoral environment that Army must learn to work around, where of prominence is the need to know structure inside, under and next to islands and coastlines. Such structures can’t be seen using existing detection techniques. Furthermore, the extended nature of the archipelago and the sheer number of islands pose a difficult reconnaissance challenge. For example, recent developments in the South China Sea show that atolls can be converted into military installations. Tracking militarisation on larger islands where structures can be concealed within headlands and underground poses a challenge.
Solving this grand challenge in surveillance and reconnaissance requires new technologies. One possible technology to assist in solving this challenge is advanced gravitational sensors. Gravity sensors measure the acceleration defect due to variations in the nearby mass density of the earth. The strengths of gravimetry include:
- Gravitational signals cannot be blocked and travel equally well through all matter and space.
- All items of physical importance have mass inherent to that item. You can degauss the metal in a vehicle to reduce its magnetic signature, but you cannot remove mass without fundamentally changing the object.
- Impossible to imitate. Magnetics, for example, can be emulated or even cancelled with electrical systems such as magnetic coils. Mass requires the replacement of an object with something of the same mass to simulate the same gravitational signal.
- Cant be jammed and is not susceptible to electrical interference, with no 50Hz mains power or ionospheric diurnal/solar noise.
- Material agnostic. Does not require ferrous or metallic objects for detection as is typical of magnetics.
- Passive sensing. It can be used to measure remote signals silently. It will not reveal sensor location like radar.
The purpose of this post is to introduce gravity sensors, their principles of operation, strengths, and weaknesses and exhibit their application through two case studies: detection of subterranean structures and detailed ocean floor mapping.
Measuring gravity
The concept of gravity is one that we are all familiar with, living bound by the gravitational attraction of our planet. The strength of the earth’s gravitational field was first measured in the 16th century by rolling a ball down an inclined plane, and the value is commonly known as an acceleration of 9.8 m.s-2. However, it is not only large bodies of mass like the planets that have a gravitational attraction. All mass generates a gravitational field. For example, there is a gravitation attraction between you and all objects around you, no matter how small, but the force of these attractions is so tiny you can’t feel them or easily detect their presence.
With sufficiently advanced sensors and instrumentation, these tiny forces can be measured and used to gain information about the density variations of the surrounding area. For example, the void a hidden underground bunker creates could be sensed as a decrease in the local gravitational field from a surface-level detector.
Gravity is a unique physical signal, and a fundamentally different force from electromagnetic signals commonly used in most detection modalities, such as imagery, radar and thermal. No known material or mechanism can block or remove the force due to gravity, and these mass signals can be sensed through any transmission medium, from vacuum to solid rock. These properties make gravity an interesting candidate for detecting hidden things.
Gravity is typically measured with a type of accelerometer known as a gravimeter, designed to have high sensitivity and be able to measure small changes in acceleration caused by density variations in the earth’s crust. These devices can routinely measure down to the shift in gravity caused by the relative position of the sun and moon as the planet rotates, known as the earth tides.
Why Now? Quantum sensor technology
So far, all of the mentioned concepts are simple and explainable, with an understanding of gravitational physics and some clever thinking about the signals you might be able to measure. However, outside of some specific mineral exploration fields targeting large mass deposits, the measurement of gravitational signals at a useful level for detecting human-scale structures has been limited to the world of research laboratories and geodetic surveys.
With the recent development in quantum technologies, new sensors that can measure minute changes in gravity with a field-deployable package are becoming closer to reality. The opportunity to use these sensors to unlock previously inaccessible detection capabilities becomes interesting.
The most common form of this new sensor is that of the cold atom freefall absolute gravimeter. These devices utilise laser cooling to create a trapped atom cloud at near absolute zero temperatures (initialisation stage). This cold atom cloud is then dropped, using it as a quantum state in freefall. The cloud is measured with laser pulses (control signals) as it falls, and the resulting atomic phase due to gravity is read out at the end of the sequence (measurement) . Devices based on this technique will likely be the next generation of absolute gravimetry sensors, replacing the old standard of a falling corner-cube laser interferometer. A key metric of these devices is their excellent accuracy (ability to measure the true value), as all critical components of the measurement, namely the clock and laser control pulses, are locked to atomically referenced systems.
On the other end of the scale, small high precision MEMS based gravimeters with optical readouts are also showing promising developments. While not absolute gravimeters like the cold atom devices, these sensors can have a much higher bandwidth and are likely to be smaller in cost, size, weight and power for the foreseeable future. Such technologies may enable supporting configurations where small MEMS-based gravimeters that can be calibrated to an absolute cold atom quantum sensor enable drone swarm gravimetry surveys.
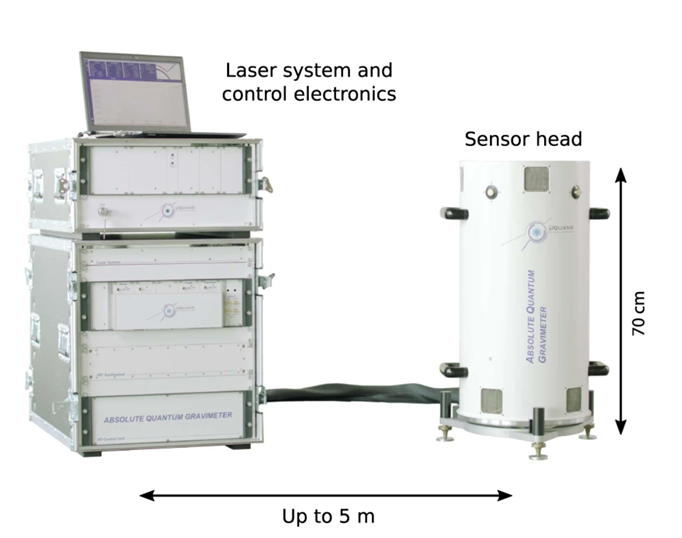
![Small form factor MEMS Gravimeter developed at the University of Glasgow [2].](/sites/default/files/small_form_factor_mems_gravimeter.jpg)
What use is it?
The full range of capabilities and applications enabled by this new generation of gravimeters is still being explored, but the basic concept always comes back to sensing a mass density variation at a distance. One of the most obvious applications in the Army context is gravimetry for detecting unobservable structures.
Case Study 1: An aerial gravity survey using gravity and gravity gradients to detect the construction of concealed facilities and fortifications in the Indo-Australian Archipelago chain. This is demonstrated in Figure 1, where a submarine pen with a hidden entrance has been built into an island. Gravimetric surveying of this island could be used to identify and track the progression of such a facility.
For gravimetry, the best signal is achieved by getting the sensor as close as possible to the target of interest. For this case study, let us take a hypothetical sensor that can measure down to 10-9 m.s-2 and assume all other platform noise and motion can be removed. Let’s take our target as a structure tunnelled out of rock with a density of 2.5 tonnes per cubic meter. The closer our gravimeter is to the structure of interest, the smaller the detectable limit becomes. Using a simple point mass approximation, the acceleration, a, due to gravity is given by,
where G is the gravitational constant, m is the object’s mass, and r is the distance of that object from the sensor. This lets us roughly equate our minimum visible target structure size in cubic meters.
Platform Altitude (meters above target) |
Minimum detectable structure (cubic meters) |
---|---|
1000 |
6000 |
100 |
60 |
10 |
0.6 |
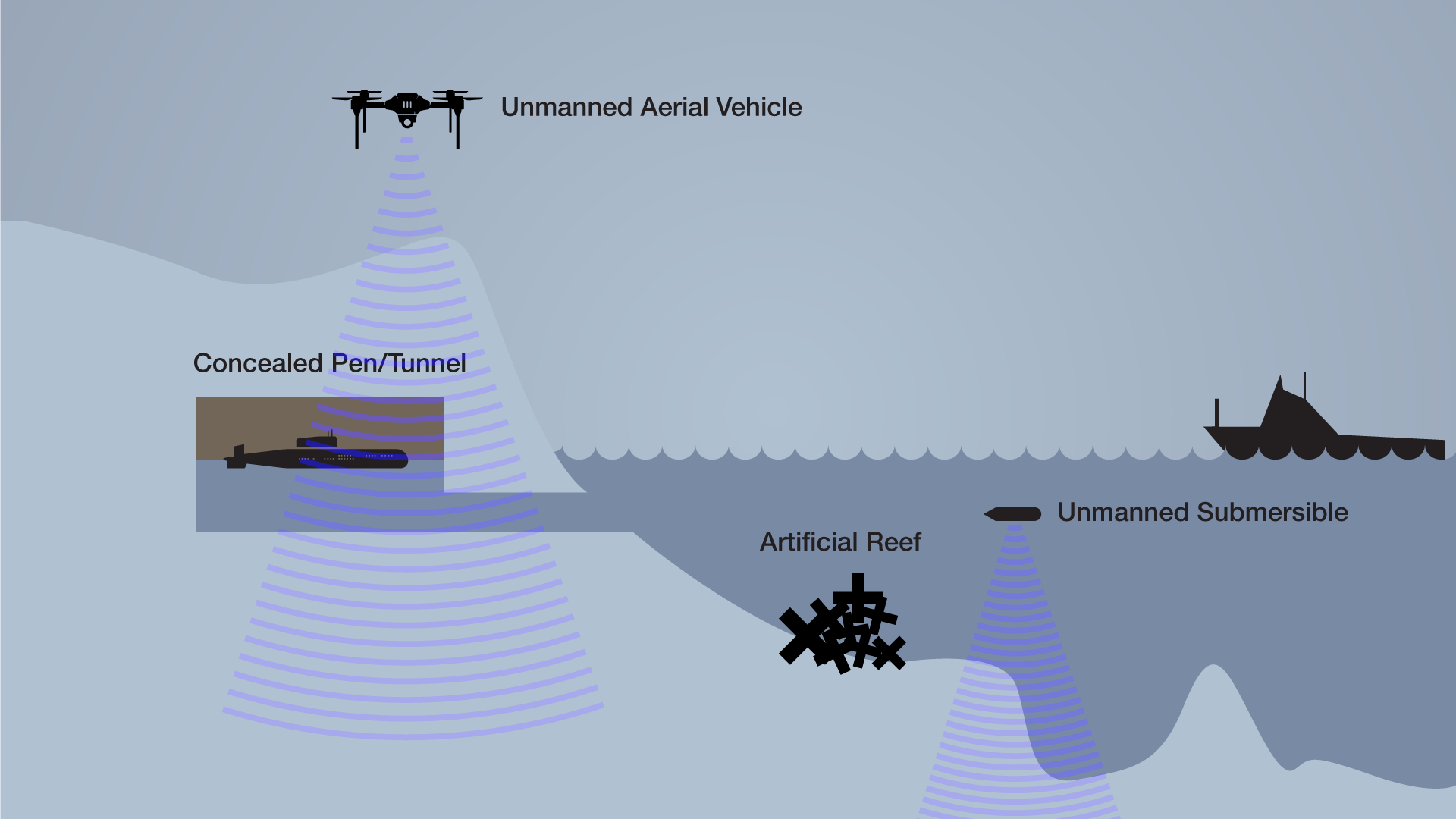
Case study 2: Autonomous underwater vehicle for detecting modification of ocean topography
The littoral environment includes another reconnaissance challenge. That is the nearshore ocean and the first 50 meters depth, where the typical visible range is less than 10 meters. An autonomous underwater vehicle could use gravimetry’s passive and silent nature to survey and map the ocean floor’s topography while remaining undetected.
A submersible platform is ideally suited to this task as it will be stable and slow-moving. These gravitational maps would aid with inertial navigation in GPS-denied scenarios or as references to observe the formation of artificial reefs and other submarine activities. While the resolution and accuracy of this technique may be inferior to a depth-sounding measurement, the advantage of making it completely undetected may be of significant use.
The simple demonstration of these detection capabilities is in itself a deterrence. The advantage of building hidden defences and facilities at considerable cost and effort will be discouraged by the ability to identify the signal from any such structure through the change in mass that it creates.
These new technologies will give the Army additional tools to gain assurance on safe landing sites and increase the maneouvre rate achievable when transitioning from sea to land in an untrusted environment.
Challenges for gravity sensing and comparison with other sensing and imaging modalities
To achieve these capabilities, several challenges need to be addressed, especially in the case of an airborne platform.
- Mixing with platform acceleration. Measured acceleration is indistinguishable from platform acceleration. Taking measurements on a moving object requires isolation or measurement and removal of that object’s accelerations.
- Background variations in the earth’s crust. The planet has a naturally varying gravitational field. Differential measurements might be needed to remove this background noise. For example, a first measurement may be required to set a baseline, and changes will only be observable from this point onwards.
- Detection of distant signals. Gravity will always suffer from the inverse square law, and unlike electromagnetic radiation, it cannot be altered or focused to give an extended range. Even if sensors are developed capable of measuring signals past 10-12 m.s-2, these signals will likely be limited by natural seismic and environmental noise.
Conclusion
In general, the motivation behind investigating gravimetry as a form of remote sensing is to complement the existing range of measurements and signatures intelligence and enhance capabilities, especially in revealing large stationary concealed targets. To progress this capability, defence needs to think through the potential use cases of gravimetry and hypothesise requirements as a goal for research and industry to aim for and iterate this process on the resulting technology.
As a first pass, much could be done with a focus on simulation to assess the feasibility and then clearly define the requirements needed to achieve use cases like the ones discussed above. Finally, in-field experimentation with existing and prototype sensors will help to build experience within defence and generate new application ideas. Tests of this type should be used to benchmark, track and foresee the technology development.
Image References:
- Ménoret, V. et al. Gravity measurements below 10−9 g with a transportable absolute quantum gravimeter. Sci Rep 8, 12300 (2018). https://doi.org/10.1038/s41598-018-30608-1
- Carbone, D. et al The NEWTON-g Gravity Imager: Toward New Paradigms for Terrain Gravimetry. Front. Earth Sci. 8:573396 (2020). https://doi.org/10.3389/feart.2020.573396
The views expressed in this article and subsequent comments are those of the author(s) and do not necessarily reflect the official policy or position of the Australian Army, the Department of Defence or the Australian Government.
Using the Contribute page you can either submit an article in response to this or register/login to make comments.